1. Introduction
The need to safeguard public health and protect the environment led to the development of sewage treatment plant. As urban areas expanded, improper disposal of wastewater resulted in pollution and widespread waterborne diseases. Recognizing these health risks spurred the creation of methods to reduce pollutants and prevent contamination. Today, a sewage treatment plant, also referred to as a domestic or municipal wastewater treatment facility plays a vital role in managing urban sewage. By eliminating harmful substances, we help preserve ecosystems and enhance community well-being. This progress reflects our growing understanding of the connection between sanitation, environmental care, and public health.
1.1 Importance and Overview
Sewage treatment is essential for purifying wastewater by removing contaminants to make it safe for discharge or reuse. It aims to produce an effluent that minimizes water pollution or can be reused effectively. Wastewater includes water from homes, businesses, and industries, often mixed with groundwater, surface water, and stormwater.
Industrial wastewater shares similarities with community sewage and can be treated with similar methods. Effective sewage treatment plants are crucial for preventing ecosystem damage and protecting public health. Untreated wastewater can lead to severe health issues and environmental disruption. Currently, about 359 billion cubic meters of wastewater are produced globally each year, with 48% released untreated. In Asia, only 32% of wastewater is treated due to inadequate facilities, financial constraints, and a shortage of qualified personnel.
Sewage treatment offers numerous benefits, with modern technology enhancing efficiency and reducing manual labor. Today’s sewage treatment plants can process large volumes of waste with less manpower and time. One key advantage is energy production through anaerobic digestion, generating methane to power the plant and nearby areas. Additionally, sludge from treatment provides nutrient-rich fertilizer ideal for agriculture. Sewage treatment reduces health risks, especially in regions like India where access to safe drinking water is limited and millions suffer from waterborne diseases. By removing contaminants, sewage treatment protects environmental health and makes water safe for reuse.
1.2 Historical Context
Before the late 1800s, outdoor privies were the norm for waste disposal. Sewage treatment systems emerged in cities after scientists like Louis Pasteur linked sewage to infectious diseases. Early methods involved spreading sewage on land, which eventually led to land degradation. Direct discharge into water bodies also worsened water quality, showing that natural systems couldn’t handle endless waste.
The evolution of wastewater treatment began with addressing environmental damage and public health concerns, initially focusing on removing solids and organic matter. By the 1970s, treatment expanded to include nutrient removal and aesthetic improvements. Since 1990, attention has turned to managing toxic chemicals and compounds with long-term health and environmental risks, resulting in advanced treatment methods. The table below outlines the key shifts in sewage treatment technologies, highlighting the progression from historical practices to modern, sustainable solutions in response to evolving environmental concerns and regulations.
Era | Historical Methods | Transition Period | Modern Advanced Treatments | Infrastructure Impact |
Early | Open-air cesspits, direct dumping | Awareness of water pollution | Primary Treatment: Sedimentation and filtration | Initial construction of basic facilities |
19th Century | Septic tanks, basic filtration | Birth of municipal sewage systems | Secondary treatment: biological processes | Expansion of sewer networks |
Mid-20th | Activated sludge, trickling filters | Wastewater treatment regulations | Tertiary treatment: advanced filtration | Upgrade to accommodate stricter standards |
Late 20th | Extended aeration, UV disinfection | Focus on nutrient removal | Advanced oxidation processes, membrane tech | Adoption of energy-efficient technologies |
21st Century | Membrane bioreactors, resource recovery | Smart technologies, data integration | Sustainable and energy-neutral solutions | Integration of smart sensors and analytics |
Table 1: Comparative table on sewage treatment evolution.
Note: The above table is made using references from studies of Niraj et al., 2011; Sahil and Premchand., 2021.
1.3 Environmental impact of sewage treatment
Sewage treatment breaks down complex organic compounds using physico-chemical methods or biological treatment with microorganisms. Releasing untreated wastewater into the environment causes severe issues like oxygen depletion, eutrophication, and contamination by harmful substances. According to UNESCO (WWAP, 2020), untreated wastewater leads to over 90% of the 1.7 million annual deaths in developing countries due to waterborne diseases like cholera and typhoid. Proper treatment eliminates disease-causing microorganisms, reduces disease vectors like mosquitoes, and prevents environmental degradation, protecting ecosystems and enhancing community quality of life.
2. Fundamentals of Sewage Treatment: Key Processes Explained
The principles of a sewage treatment plant are crucial for effective and sustainable wastewater management, ensuring the protection of both the environment and public health. The process involves transforming sewage into treated water that meets regulatory standards through a series of physical, biological, and chemical treatments designed to remove pollutants and impurities. This overview highlights the core components of a sewage treatment plant, from initial screening to final effluent disposal or reuse, focusing on the processes, infrastructure, and environmental considerations necessary for efficient wastewater treatment.
2.1 What Defines a Sewage Treatment Plant: Essential Features
A sewage treatment plant is a specialized plant designed to receive and process wastewater from households, industries, and businesses. Its main goal is to remove contaminants and pollutants, ensuring the treated water meets environmental and regulatory standards before being discharged into natural water bodies or reused for non-potable purposes. The treatment process addresses various pollutants, including solids, organic carbon, nutrients, inorganic salts, metals, and pathogens (Sahil and Premchand, 2021). This purification involves a combination of physical, biological, and chemical methods to safeguard public health and reduce the environmental impact of wastewater discharge. A typical sewage treatment facility operates by receiving raw sewage, screening out large debris, performing primary and secondary processes to remove impurities, disinfecting to kill pathogens, managing sludge, and producing treated water for safe disposal or reuse, all while adhering to environmental standards with continuous monitoring. State-wise wastewater generation and treatment capacity are illustrated in Fig 1.
Fig 1: Venn diagram depicting Sewage generation, Installed treatment capacity, Operational Capacity, Actual Utilization and Compiled Treatment Capacity (CPCB., 2021).
A sewage treatment plant (STP) involves several critical processes for effective wastewater treatment. It starts with screening to remove large debris from raw sewage. In primary treatment, sedimentation removes suspended solids, generating sludge, while the clarified water proceeds to secondary treatment. Secondary treatment uses biological processes where microorganisms break down biodegradable materials, reducing biological and chemical oxygen demand (Ayhan et al., 2017). The water then undergoes tertiary treatment with advanced filtration or chemical processes to enhance its quality. Disinfection is applied to remove pathogens, ensuring the water meets safety standards. Simultaneously, sludge is treated through digestion and dewatering for proper disposal or reuse. The final effluent is either discharged into water bodies or repurposed for non-potable uses. Efficient STP operation relies on continuous monitoring, infrastructure maintenance, and compliance with environmental regulations.
2.2 Why Sewage Treatment Matters to Everyone
A sewage treatment plant is essential for managing domestic and commercial waste, removing harmful materials to protect public health. Its goal is to produce environmentally safe treated effluent (liquid waste) and treated sludge (solid waste) that can be safely disposed of or reused, often as farm fertilizer (Maaz et al., 2017). Treated wastewater (TWW) is typically discharged into watercourses or used for irrigating parks, lawns, and public areas. However, its reuse for non-potable purposes like crop irrigation, industrial processes, and groundwater recharge remains limited. Only a small portion of treated wastewater is redirected for productive use, representing a missed opportunity to address water scarcity concerns (Niti Ayog, 2013).
Fig 2: Major waterborne diseases (Kazi et al., 2015).
Wastewater treatment is vital for protecting ecosystems and public health. Without treatment, wastewater can devastate environments and public health. Over 80% of wastewater is untreated in many developing countries, causing widespread illness and disrupting the food chain. Untreated wastewater is linked to 1.7 million annual deaths, with over 90% in underdeveloped regions. Proper sewage treatment prevents water pollution, maintains ecosystem health, and avoids issues like oxygen-depleted “dead zones” and toxic effects on wildlife and humans. It also helps prevent red tides that harm aquatic life and make water unsafe. Economically, treating wastewater saves on pollution management and healthcare costs while supporting industries reliant on clean water. Effective treatment is essential for a sustainable and healthy environment.
Explore Wastewater Treatment Solutions
Find top machinery, plants, tools, resources, companies, and consultancy for comprehensive sewage treatment plant needs.
3. Exploring Different Types of Sewage Treatment
Sewage treatment refers to a procedure that can clean up pollutants from both industrial and domestic wastewater. The four main techniques used to treat sewage water are chemical, biological, physical, and sludge treatment. Using these techniques results in treated water that is safe for use by humans and the environment, disinfecting the wastewater of all sewage contaminants.
3.1 Primary (Physical) Sewage Treatment
Primary sewage treatment is the first stage in the wastewater treatment process, focusing on preliminary treatment to remove coarse solids and large materials from untreated wastewater. This phase targets the elimination of sizable solids, including wood, cloth, paper, plastics, and garbage, as well as heavy inorganic solids like sand, gravel, metal, and glass, collectively known as “grit.” It also addresses excessive oils and greases.The primary goal is to remove or reduce these solids through physical processes such as sedimentation and flotation (Amit and Rupali, 2004). This stage typically removes about 25–50% of incoming biochemical oxygen demand (BOD), 50–70% of total suspended solids (SS), and 65% of oil and grease. Additionally, the sedimentation process forms sludge by separating settled solids, which reduces the organic load and helps protect downstream biological treatment processes.
Fig 3: Comparison of removal efficiency of COD by primary sedimentation of raw wastewater during individual treatment stages using stainless steel (SS), iron (Fe), and aluminum (Al) electrodes, and primary sedimentation of a mixture of raw wastewater with previously generated sludge during individual treatment stages using stainless steel (SS), iron (Fe), and aluminum (Al) electrodes (Morana et al., 2022)
While some organic nitrogen, organic phosphorus, and heavy metals associated with solids are also eliminated during primary sedimentation, colloidal and dissolved constituents remain unaffected. The resulting effluent from primary sedimentation units is termed primary effluent. The principal function of primary treatment is to act as a precursor to secondary treatment (McGhee., 1991). Table 2 provides information on primary effluent from three sewage treatment plants in California along with data on the raw wastewaters.
Table 2: Quality of raw wastewater and primary effluent at selected treatment plants in California (Amit and Rupali., 2004).
While primary treatment is effective for physical pollutant removal, it has limitations. It does not effectively remove dissolved pollutants or colloidal matter in the wastewater. Although primary treatment removes a portion of organic matter, it does not achieve the same level of reduction as secondary and tertiary treatments, which are essential for advanced organic pollutant removal. It also has limited impact on nutrient removal, including nitrogen and phosphorus compounds, which are crucial contributors to water pollution and are thus followed by more advanced treatment processes.
3.2 Secondary (Biological) Sewage treatment options
Secondary sewage treatment follows primary treatment to further purify the effluent by removing remaining organics and suspended solids (Amit and Rupali, 2004). Unlike primary treatment, which focuses on physically removing larger solids, secondary treatment emphasizes the use of microorganisms to biologically break down organic pollutants. During this phase, bacteria decompose fine organic matter in aerobic or anaerobic conditions, producing a clearer effluent (Henze and Harremoes, 1983). This process, known as biological treatment, utilizes microorganisms to further treat wastewater, effectively removing suspended solids and biodegradable organics.
Fig 4: % Reduction in BOD and COD after secondary treatment (Rana et al., 2019).
Secondary sewage treatment relies on biological processes to further purify wastewater by removing residual organic pollutants. These processes are categorized based on the oxygen availability and the metabolic pathways of microorganisms, including aerobic, anoxic, and anaerobic (Shaikh et al., 2013). Key biological treatment methods include:
- Activated Sludge Process: Introduced in the early 1900s, this method involves aerating wastewater to encourage microorganisms, or “activated sludge,” to consume organic matter. The mixture of wastewater and microorganisms, known as “mixed liquor,” undergoes sedimentation to separate the treated water from the sludge (Shaikh et al., 2013).
- Trickling Filter Process: This aerobic system uses microorganisms on a medium, such as rocks or plastic media, to treat wastewater as it trickles over the surface. It’s commonly used in technologies like rotating biological contactors and packed bed reactors (EPA, 2000).
- Rotating Biological Contactors (RBC): Developed in the 1970s, RBCs feature rotating discs with a microbial biofilm that treats wastewater as it rotates through it, improving biological treatment efficiency (Singh and Mittal, 2012).
- Sequential Batch Reactor (SBR): This system treats wastewater in a single tank through sequential phases—filling, aerating, settling, and decanting—providing flexibility in treatment processes.
- Membrane Bioreactors (MBR): MBRs combine membrane filtration with biological treatment to produce high-quality effluent by retaining microorganisms in the reactor.
- Constructed Wetlands: Using soil, microbes, and plants, this method naturally cleans wastewater and improves nitrogen removal through eco-friendly processes.
- Oxidation Ditches: These systems promote biological treatment by aerating and mixing wastewater, allowing microorganisms to break down contaminants over a longer detention period.
- Biological Aerated Filter (BAF): BAF systems use filter media combined with aerobic microorganisms to remove organic materials from wastewater as it flows through the filter bed.
- Hybrid Systems: These systems combine various biological treatment methods to leverage their strengths and achieve optimal treatment efficiency.
Table3: Comparison of performance after Secondary Treatment of major technologies (Tare V., 2010).
Studies show that Membrane Bioreactor (MBR) technology excels in removing Biochemical Oxygen Demand (BOD) and Suspended Solids (SS), achieving levels below 5 mg/l (Harpreet and Sanjay., 2022). Secondary sewage treatment is crucial for reducing pollutants and nutrients to ensure safe effluent discharge or reuse. The choice of method depends on treatment goals, space, and regulations.
Challenges include managing sludge and varying influent quality. Modern solutions involve advanced sludge treatment technologies like anaerobic digestion and real-time process control, with smart technologies enhancing efficiency and adaptability.
3.3 The Role of Tertiary (Advanced) Sewage Treatment in Water Purification
Tertiary treatment is the final stage of wastewater treatment, designed to further enhance water quality after primary and secondary treatments (Ayhan et al., 2017). This phase aims to remove residual contaminants, including dissolved pollutants, fine particles, and pathogens, ensuring the treated water meets stringent regulatory standards for safe discharge or reuse (Maaz et al., 2017). Tertiary treatment employs several advanced methods:
- Filtration: Advanced filtration techniques, such as sand, multimedia, or membrane filters, capture fine suspended particles, bacteria, and remaining solids, improving water clarity.
- Chemical Treatment: Methods like coagulation, flocculation, and advanced oxidation target dissolved pollutants and pathogens, facilitating their removal or breakdown.
- Nutrient Removal: This process focuses on removing nutrients like nitrogen and phosphorus to prevent issues like algae blooms in receiving waters.
- Disinfection: Various disinfection methods ensure the elimination of pathogens:
- Chlorination: This widely used method involves chlorine reacting with organic compounds, though it can form harmful byproducts and does not affect nutrient content (Albolafio et al., 2022).
- Ultraviolet Irradiation: UV light disrupts the DNA and RNA of pathogens, providing effective and chemical-free disinfection (Dimitris and Georgios., 2022).
- Ozonation: Ozone, a powerful oxidizer, disinfects water by reacting with contaminants and microorganisms. It is effective against bacteria, viruses, and protozoan cysts and helps remove taste, odor, and color (Leonardo et al., 2009).
Tertiary treatment is crucial for protecting the environment and ensuring that water can be safely released into natural bodies or repurposed, especially in areas with limited water resources.
The following table presents the CPCB evaluation before and after tertiary treatment alongside DEWATS (Decentralized Wastewater Treatment System), which follows a concept with low cost, O&M and energy intensive nature-based systems, mostly composed of anaerobic treatment and extended planted gravel filtration (Schellenberg et al., 2020)..
Table 4: Removal efficiency of various pollutants before and after tertiary treatment.
The goal of tertiary treatment is to provide effluent that satisfies strict water quality criteria so that it can be used for potable water reuse or directly discharged into ecosystems that are sensitive to it. Tertiary sewage treatment makes a major contribution to sustainable water management practices and environmental preservation by optimizing the quality of treated water by removing remaining contaminants.
3.4. Specialized Sewage Treatment Methods: Quaternary Treatments and Beyond
Quaternary sewage treatment is an advanced stage of wastewater purification aimed at removing emerging contaminants that conventional treatments (primary, secondary, and tertiary) often miss. Micropollutants, such as pharmaceuticals, household chemicals, environmentally persistent pharmaceutical pollutants (EPPP), and pesticides, can persist through traditional processes, contributing to water pollution. Quaternary treatment utilizes specialized technologies to target these challenging micropollutants. However, the high cost of these techniques limits their widespread adoption (Ayhan, 2017). This treatment represents the forefront of wastewater purification, enhancing water quality and environmental safety.
Fig 5: % removal of micro-pollutants by quaternary treatment techniques (Biniam et al., 2023).
Quaternary sewage treatment employs specialized techniques to address contaminants that traditional methods may miss, such as pharmaceuticals and micropollutants. Here’s a look at the advanced methods used:
- Advanced Oxidation Processes (AOPs): These methods, including ozonation, photocatalysis, and Fenton’s reagent, enhance purification by targeting pharmaceuticals and persistent organic pollutants beyond conventional treatments.
- Membrane Technologies: Techniques like reverse osmosis and nanofiltration offer high-quality water by removing dissolved impurities, crucial for applications requiring very pure water (Amit and Rupali, 2004).
- Electrochemical Treatment: Methods such as electrooxidation and electrocoagulation use electrical currents to remove contaminants, metals, and microbes, providing a sustainable approach to water treatment.
- Constructed Wetlands with Engineered Components: This approach combines natural processes with engineered systems to enhance nutrient removal and provide an eco-friendly treatment option (Vymazal, 2010).
- Ultraviolet (UV) Advanced Oxidation: UV irradiation, combined with advanced oxidation, effectively breaks down pathogens and organic contaminants for thorough water cleaning.
- Biological Nutrient Removal (BNR): BNR processes use advanced biological techniques to selectively remove nutrients like nitrogen and phosphorus, addressing eutrophication in water bodies.
- Membrane Bioreactors (MBRs): Combining biological treatment with membrane filtration, MBRs improve solid-liquid separation and produce high-quality effluent suitable for various reuse applications.
These advanced methods represent the forefront of wastewater treatment, targeting challenging contaminants and promoting sustainable practices like water recycling and zero liquid discharge.
Adsorbent | Target Metal | pH | Initial Metal Concentration (mg/L) | Contact Time (min) | Adsorption Capacity (mg/g) | RemovalPercentage (%) | |
Activated carbon from African palm fruit | Cd | 8 | 1820.00 | 60 | N/A | 99.23 | |
Cu | 3 | 1520.00 | 60 | N/A | 96.71 | ||
Ni | 8 | 3240.00 | 60 | N/A | 95.34 | ||
Pb | 3 | 2620.00 | 60 | N/A | 97.75 | ||
Magnetic graphene oxide | Pb | 5 | 60.00 | 25 | 200.00 | 89.61 | |
Cr | 6 | 60.00 | 35 | 24.330 | 92.03 | ||
Cu | 6 | 60.00 | 25 | 62.89 | 92.43 | ||
Zn | 7 | 60.00 | 35 | 63.69 | 90.38 | ||
Ni | 8 | 60.00 | 25 | 51.02 | 92.23 | ||
Silica oxide encapsulated natural zeolite | Pb | N/A | 10.00 | 30 | 186.00 | 99.30 | |
Cu | N/A | 10.00 | 480 | 10.30 | 98.90 | ||
Cd | N/A | 10.00 | 60 | 12.30 | 98.30 | ||
Zn | N/A | 10.00 | 60 | 9.00 | 97.10 | ||
Mn | N/A | 10.00 | 30 | 4.20 | 54.00 | ||
Plasma polymer functionalized silica | Cu | 5.5 | 15.00 | 60 | 25.00 | >96.70 | |
Zn | 5.5 | 15.00 | 60 | 27.40 | >96.70 | ||
Polyaniline grafted cross-linked chitosan beads | Cd | 6 | 40.00 | 60 | 145.00 | 99.60 | |
Pb | 5 | 40.00 | 60 | 114.00 | 99.30 |
Remarks: N/A is no data available.
Table 5: The removal efficiency of several commonly used adsorbents toward heavy metals (Muhammad et al., 2021).
Most functionalized adsorbents have an excellent adsorption ability toward targeted heavy metals (>90%), when enhanced with optimum pH condition, contact time and concentration.
These quaternary and beyond speciality treatments are designed to fulfill particular water quality goals, deal with new pollutants, and offer long-term wastewater management solutions. By using these cutting-edge techniques, sewage treatment is ensured to be more comprehensive and focused, promoting environmental sustainability and water resource management.
Struggling with sewage treatment plant issues? Connect with top consultants specializing in sewage treatment plant solutions.
Connect Now4. In-Depth Analysis of Sewage Treatment Technologies
Sewage treatment, also known as domestic or municipal wastewater treatment, is a process designed to eliminate impurities from sewage. The goal is to generate treated water, known as effluent, that meets the standards for discharge into the environment or reuse applications. This process serves to safeguard against water pollution resulting from the release of untreated sewage into the environment. An in-depth analysis of sewage treatment technologies involves a comprehensive examination of various methods employed in the purification of wastewater.
4.1 The Mechanics of Physical Treatment: Screening and Sedimentation
In the realm of physical wastewater treatment, the mechanics of screening and the sedimentation process are integral components of the initial stages, particularly in primary sewage treatment techniques. These processes are paramount for the removal of large solids and the separation of suspended particles in sewage.
Screening in sewage treatment: Screening is a vital component of physical wastewater treatment, utilizing different types such as bar screens, rotating drum screens, and fine screens to remove larger debris like sticks and plastics. This process helps protect downstream equipment, preventing damage to pumps and pipes, and ensuring efficient system operation.
Table 6: Presents efficiency of screening (various sizes of solids removal from wastewater) (US EPA., 2003).
Grit removal in sewage treatment: Grit removal is a key step in physical wastewater treatment, working alongside the screening process to remove larger particles like sand and gravel. This separation of grit prevents abrasion and damage to downstream equipment, enhancing the efficiency and longevity of the sewage treatment plant.
Sedimentation process in wastewater treatment: Sedimentation, a key component of wastewater treatment, uses tanks to separate suspended solids by gravity. As wastewater sits in these tanks, heavier particles settle into a sludge layer at the bottom, while the lighter, clarified liquid remains on top. This process reduces solid content efficiently, setting the stage for biological and advanced treatment methods that follow.
Fig 6: Efficiency of various physical treatment methods.
Understanding the intricacies of screening and the sedimentation process is fundamental to optimizing physical wastewater treatment. These primary sewage treatment techniques set the stage for the comprehensive removal of contaminants, emphasizing the importance of efficient grit removal and the role of sedimentation tanks in achieving high-quality treated effluent.
4.2. Biological Treatment Unveiled: Aerobic vs. Anaerobic Processes
Biological treatment is essential in sewage treatment plants, using microorganisms to break down organic pollutants. Unlike chemical methods, which are costly and produce hard-to-dispose sludge, biological treatment is more sustainable. The activated sludge process is a key method where microorganisms in aerated tanks digest organic pollutants. This process not only reduces waste but can also generate biogas through anaerobic digestion, promoting sustainable energy. Biological treatment also helps with nutrient removal, preventing eutrophication in water bodies. There are two main types: aerobic, where microorganisms need oxygen, and anaerobic, which produces methane in the absence of oxygen. Both are vital for effective wastewater management in different types of sewage treatment plants.
Aerobic treatment:
Aerobic treatment in sewage plants relies on aerobic bacteria to break down organic pollutants in the presence of oxygen. These bacteria use oxygen to decompose organic matter, transforming it into simpler substances like carbon dioxide, water, and microbial biomass. This process significantly reduces the biochemical oxygen demand (BOD) of the wastewater. Common aerobic systems include the activated sludge process, where wastewater is mixed with microbial cultures in aerated tanks, and trickling filters, where water flows over a media bed promoting microbial growth. Additionally, rotating biological contactors use rotating discs that support bacterial growth, enhancing the treatment process by maximizing the contact between wastewater and bacteria.
Anaerobic Treatment:
When we talk about anaerobic treatment, we refer to processes that occur without the presence of oxygen, leading to sulfate reduction and methanogenesis. In these systems, anaerobic bacteria break down complex organic compounds through anaerobic digestion, producing methane (CH₄) as a valuable renewable energy source. This method not only generates useful biogas but also results in lower amounts of biosolids. By leveraging anaerobic digestion, we effectively manage wastewater with reduced environmental impact.
Fig 7: Comparison chart showing aerobic and anaerobic processes (Gasparikova et al., 2005).
Common Anaerobic Treatment Systems:
- Anaerobic Digesters: In anaerobic digesters, organic-rich wastewater is processed in sealed tanks without oxygen. This environment supports methane-producing bacteria, leading to biogas generation and a residual sludge. The biogas is a valuable energy source, and the sludge can be further treated or used as a soil conditioner.
- Upflow Anaerobic Sludge Blanket (UASB) Reactors: UASB reactors involve wastewater flowing upwards through a suspended sludge bed, ideal for anaerobic bacteria. This process efficiently converts organic matter into methane-rich biogas, and the treated effluent is clarified for reuse or discharge. Compared to aerobic stabilization, UASB treatment is more energy-efficient, handles higher loading rates, and produces less sludge with improved dewaterability (Lim and Kim., 2014).
Comparing Aerobic and Anaerobic Processes:
Efficiency:
- Aerobic: Aerobic processes excel at removing organic pollutants, leading to lower biochemical oxygen demand (BOD) levels in the treated water.
- Anaerobic: While anaerobic processes are effective in decomposing organic matter, they might not achieve the same BOD reduction as aerobic methods.
Speed of Treatment:
- Aerobic: Aerobic treatment is typically faster, thanks to the rapid metabolic activities of aerobic bacteria.
- Anaerobic: Anaerobic treatment tends to be slower, requiring longer retention times to effectively digest organic matter.
Energy Consumption:
- Aerobic: Aerobic systems such as activated sludge or trickling filters have higher energy demands due to the need for aeration.
- Anaerobic: Anaerobic digestion consumes less energy and produces biogas, a renewable energy source.
End Products:
- Aerobic: Breaks down organic matter into carbon dioxide and water.
- Anaerobic: Produces methane (biogas), carbon dioxide, and residual sludge.
Table 7: Characteristics of aerobic and anaerobic treatment (Amin et al., 2019).
4.3 Chemical Treatment in Sewage Plants: Key Processes and Purposes
Chemical treatment plays a crucial role in sewage treatment plants, removing contaminants left after physical and biological processes to ensure thorough wastewater purification and regulatory compliance. Chlorination disinfects wastewater, preventing waterborne diseases and maintaining effluent quality. Phosphorus removal prevents eutrophication, reducing environmental impact. Disinfection eliminates harmful microbes, enhancing water safety for reuse or discharge. Chemical treatments also reduce Chemical Oxygen Demand (COD), improving efficiency. Advanced methods like flocculation, coagulation, and pH adjustment use chemicals like alum and ferric chloride to aggregate particles for easier removal.
Chemical | Purpose | Efficacy (% Reduction) |
Chlorine | Disinfection | Varied (typically high) |
Ferric Chloride | Phosphorus removal | Up to 90% |
Polyacrylamide | Flocculation | Enhances Sedimentation by 70% |
Sodium Bisulfite | Dechlorination | Variable |
Lime (Calcium Hydroxide) | pH adjustment,phosphorus removal | Depends on dosage |
Ozone | Disinfection, COD reduction | Depends on dosage |
Despite its benefits, chemical treatment faces challenges such as the need for strict safety protocols and training to prevent accidents or exposure. Some chemicals can produce by-products, like disinfection by-products (DBPs), requiring extra treatment and posing regulatory issues. Residual chemicals may also affect the environment, necessitating careful management to reduce ecological impact and ensure compliance. Moreover, the procurement and disposal of chemicals increase operational costs, requiring regular monitoring and optimization to maintain cost-effectiveness.
Treatment Method | Advantages | Disadvantages |
Chlorination | Widely available | Formation of disinfection by-products |
Ozonation | Effective against a wide range of pathogens | Higher cost compared to other methods |
UV radiation | Chemical free process, no residual chemicals in water | Less effective on certain types of microorganisms |
Advanced Oxidation Processes | Highly efficient at degrading organic matter and eliminating pathogens | Complex system design and operation required |
4.4 Innovations in Tertiary Treatment: Membrane Filtration & UV Disinfection
Membrane filtration is a powerful method that filters out impurities and particles based on their size and molecular composition while allowing water molecules to pass through. Water is pushed through membranes under pressure, with different types of membranes designed for various filtration needs:
- Microfiltration (MF): These membranes have a pore size around 1 μm and effectively remove suspended solids.
- Ultrafiltration (UF): With smaller pores than MF, UF membranes reject larger dissolved molecules.
- Nanofiltration (NF): These membranes, with pores around 1 nm, can filter out smaller dissolved molecules (up to 200 Da) and divalent ions.
- Reverse Osmosis (RO): RO uses a membrane without pores, relying on diffusion rates of solutes to achieve separation (Dimitris and Georgios, 2022).
This method is essential for producing high-quality water by efficiently removing contaminants based on their size and molecular characteristics.
Heavy Metal | Process | % Removal |
Arsenic (As) | UF | 83.65 |
Cadmium (Cd) | UF | 79.1 |
Chromium (Cr)Copper (Cu) | NFNF | 96-9995.33 |
Lead (Pb) | UF | 90.8 |
Nickel (Ni) | NF | 94.99 |
UV disinfection uses ultraviolet (UV) light (around 254 nm) to inactivate microorganisms in wastewater. UV light disrupts the DNA or RNA of pathogens, including bacteria, viruses, and protozoa, preventing their reproduction and ensuring they are inactive.
Benefits:
- Chemical-Free: No residuals left in water.
- Effective: Inactivates a wide range of microorganisms.
Challenges:
- High Initial Costs: Significant investment required.
- Energy Consumption: Requires substantial energy.
- Maintenance: Regular upkeep needed.
- Turbidity Impact: Less effective in turbid water, where suspended solids can block UV light.
Find and connect with leading companies specializing in sewage treatment solutions.
Get Connected Today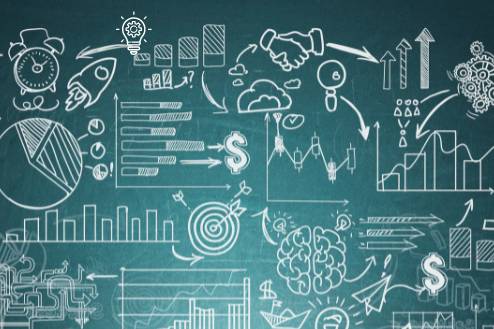
5. Choosing the Right Sewage Treatment Plant for Your Needs
The challenge in wastewater management is selection of the best available technology for the particular wastewater treatment objective at a particular site. Many factors, such as capital costs, operation and maintenance (O&M) costs, and land requirements, are involved in the decision-making process (Pradip et al., 2012).
5.1 Criteria for Selecting the Best Sewage Treatment Plant
When selecting a sewage treatment plant, start by evaluating the wastewater volume to ensure the plant meets current and future needs. Consider the environmental impact of the technology, favoring energy-efficient and sustainable options. Balance initial costs, operational expenses, and maintenance to find a cost-effective solution. Compare different sewage treatment technologies to find the best fit for your wastewater and required effluent quality. Finally, ensure the plant complies with all local, regional, and national regulations to avoid legal issues and ensure effective wastewater management.
Fig 9: Comparison of O&M cost of various technologies for STPs; Table 10: Comparison of Operation & Maintenance cost of various technologies for STP (Tare V., 2010)
5.2 Budget Considerations in Sewage Treatment Plant Selection
Choosing a sewage treatment plant involves careful financial planning to balance cost-effectiveness and long-term sustainability. We need to evaluate both initial and ongoing costs, ensuring the plant is affordable and efficient. Upfront expenses include purchasing property, construction, and equipment, which can vary based on the plant’s capacity and technology. Typically, more advanced technologies and larger capacities come with higher initial costs. Striking the right balance between economic viability and environmental sustainability is crucial for effective wastewater management.
Table 11: Comparison of area requirement and treatment costs of various technologies for STP; Fig 11 : Comparison of treatment costs of various
technologies for STP; Fig: Comparison of area requirements of various technologies for STP(Tare V., 2010)
The sustainability of a sewage treatment plant hinges on managing ongoing costs, including energy use, chemical consumption, sludge disposal, and maintenance. Choosing the right technology is essential for long-term affordability. We should explore cost-recovery options, like selling treated water or generating electricity from anaerobic digestion. Funding strategies, including government grants, loans, and private investment, are crucial for financial feasibility and project success. Conducting thorough economic analyses is vital. Tools such as Net Present Value (NPV), Life Cycle Cost Analysis (LCCA), and Return on Investment (ROI) help evaluate financial viability. NPV assesses profitability over time, ROI measures returns on the initial investment, and LCCA evaluates total costs throughout the technology’s lifecycle. Utilizing these tools ensures informed decision-making and contributes to cost-effective, sustainable sewage treatment operations.
5.3 Environmental & Regulatory Impacts on Sewage Treatment Choices
Environmental regulations are vital in shaping responsible wastewater management and guiding sewage treatment operations. These laws enforce strict wastewater discharge guidelines at national and global levels, promoting compliance with local standards and collective conservation efforts. Regulations drive the adoption of advanced sewage treatment technologies, encouraging innovation that meets or exceeds environmental requirements, balancing effective management with ecological protection. For detailed water quality improvements before and after treatment, see the study by Geeta et al., 2021, in the table below.
Parameter | Before Treatment(Sample 1) | After Treatment(Sample 1) | Before Treatment(Sample 2) | After Treatment(Sample 2 ) |
Color | Dry white color | Colorless | Grey | Colorless |
Temperature (deg celsius) | 20 | 20 | 20 | 20 |
pH | 7.15 | 7.30 | 1.43 | 6.81 |
Total Hardness (ppm) | 1240 | 160 | 770 | 130 |
Total Dissolved Solids (ppm) | 646 | 149 | 232 | 124 |
Turbidity NTU | 30 | 2.61 | 50 | 1.77 |
Dissolved Oxygen | 6.4 | 9.6 | 0.97 | 4.5 |
Chemical Oxygen Demand | 80 | 20 | 160 | 20 |
Phosphate Test | Positive | Negative | – | – |
Sample 1. Sample 2. Sample 1. Sample 2
Sample 1 Sample 2 Sample 1 Sample 2
Fig 12: Graph showing the results of water quality parameters before and after treatment. a. Dissolved oxygen (ppm), b. chemical oxygen demand (ppm), c. turbidity (NTU) and d. total dissolved solid (ppm) (Geeta et al., 2021).
The Royal Commission on Sewage Disposal (1898-1915) set initial standards for Biochemical Oxygen Demand (BOD) at 20 mg/l and suspended solids (TSS) at 30 mg/l in treated wastewater, later updated by the Water Act of 1973 and the Urban Waste Water Treatment Directive (Johnstone and Horan, 1996). Today, the choice of sewage treatment technology depends on the desired quality of treated wastewater. Best Available Technology (BAT) is used in the Global North for cost efficiency, while Best Practicable Technology (BPT) is applied in the Global South. The ALARA principle guides risk management (CPCB, 2009), and the interaction between evolving standards and technology drives continuous innovation in sewage treatment and environmental protection.

Explore the best sewage treatment plant solutions for your industrial needs.
Connect Today6. Case Studies and Real-World Applications
Success Stories: Implementing Effective Sewage Treatment Solutions
Okhla Sewage Treatment Plant, Delhi, India:
Delhi’s Okhla Sewage Treatment Plant, with a capacity of 30 MGD, was established under the Yamuna Action Plan-II. It utilizes the Activated Sludge Process and features a biogas system for electricity generation from sludge. Fully automated and controlled by SCADA, the plant cost approximately Rs 149.5 crore (Shreya et al., 2018).
Delawas Sewage Treatment Plant, Jaipur, India:
The Delawas STP operates using the Activated Sludge Process (ASP), divided into two phases, each with a capacity of 62.5 MLD. Unique in that it requires no chemicals or pumps for sewage transport over a 25-kilometer radius, this plant produces biogas to cover 75%-80% of its energy needs. The treated water benefits farmers by improving crop yields and can be used for groundwater recharge and horticulture. The waste-to-energy approach is notable, contributing to reduced power consumption for irrigation and tree planting (Rahul, 2017).
7. The Future of Sewage Treatment
The future of sewage treatment is evolving towards sustainability, efficiency, and innovation. One major trend is the adoption of circular economy principles, where treated sewage is repurposed for water reuse, fertilizer recovery, and energy production. Modular and decentralized systems offer flexibility, while smart technologies like IoT and AI enhance treatment processes. Environmental impact is reduced through energy-neutral plants, biotechnological advancements, and nanotechnology for pollution control. Resource recovery remains a key focus, turning sewage into valuable resources. Future developments will also emphasize community engagement and climate-resilient infrastructure to promote water conservation and sustainability.
7.1. Emerging Trends in Sewage Treatment Technology
Following statistics gives us an overview on the pollution removal of TSS, NO3, BOD and COD compared between effluent water and raw sewage in the case of Kermanshah wastewater treatment plant in Iran. This study concluded that the removal efficiencies for the TSS, NO3, BOD and COD is estimated by 80-92%.
Fig 13: The removal of pollution of TSS, NO3, BOD, and COD compared between effluent water and raw sewage values (Siamak and Mohammad., 2019).
Table 13: Comparison between different sewage treatment technologies in terms of plant performance and economic factors. The information shows that stabilization ponds are the preferred wastewater treatment process in developing countries (Arthur.1983). (Key: FC = Fecal coliforms; SS = Suspended solids; G = Good; F = Fair; P = Poor).
Despite advances in current technologies, there’s always room for improvement in wastewater treatment. Strict legislation and substantial fines for non-compliance with discharge limits have driven the development of new or enhanced treatment technologies (Kwaku et al., 2020). Modern sewage treatment focuses on sustainability, aiming to reduce environmental impact through energy-efficient processes, resource recovery, and green infrastructure. Innovations in treatment methods enhance overall efficiency, optimize chemical use, and employ advanced filtration techniques to streamline operations, lower energy consumption, and ensure effective contaminant removal.
- Integration of Smart Solutions: Smart technology is revolutionizing sewage treatment with automation, real-time monitoring, and data analytics, enhancing decision-making and resource use.
- Decentralized Treatment Systems: These systems offer flexibility and resilience by reducing reliance on central infrastructure, supporting localized wastewater management.
- Advanced Membrane Technologies: Membrane technologies like microfiltration, ultrafiltration, and reverse osmosis are improving solids separation, water quality, and reuse options, enhancing sustainability.
- Emerging Biological Treatment Innovations: Innovations such as new microbial strains, bio-augmentation, and microbial fuel cells (MFCs) are advancing biological treatment. Microalgae and MFCs improve contaminant removal, energy production, and sustainability.
- Incorporation of Artificial Intelligence (AI): AI is increasingly used for monitoring and optimizing sewage treatment processes, including efficiency, BOD, COD, and contaminant prediction.
Sewage treatment technology is evolving rapidly, driven by a commitment to environmental responsibility, operational excellence, and sustainable solutions, addressing global wastewater management challenges.
7.2. Sustainable Practices in Sewage Treatment: A Green Revolution
Sustainable sewage treatment involves adopting practices that prioritize resource recovery, energy efficiency, and the reduction of carbon footprints.
Fig 14: Represents the eco-efficiency scores of various STPs based on their GHG savings, operating cost savings reduction in COD, SS, N and P. There are only four WWTPs whose eco-efficiency score is one which means they are eco-efficient (Alexandros and Maria., 2023).
The process | Environment | Economics | Social | Challenges |
Activated sludge | Low land requirementLess flies compared to waste stabilization ponds and constructed wetlands. | Cost-effective technologySkilled supervision | The most traditional and applied processNeed skilled laborsGood quality of effluent | Not flexible methodSensitive to certain industrial wastes |
Trickling filter | Good airBiological inertnessNuisance from odor is high | Low costHigh surface area per unit volume | Need skilled labors | Sensitive to high organic and volumetric loads |
Membrane bioreactor | Lower sludge production | High energy cost reduction in plant footprintHigh operation and capital costs of the membranesSecondary and tertiary filtration processes are eliminated | High efficiencyPublic awareness is neededNeed skilled labors | Membrane complexityFouling |
Adsorption | Recycling of the adsorbent is significantNuisance from odor is low | Still on the small scaleLow energy is requiredLow maintenance | Public awareness is neededNeed skilled labors | Not suitable for large volumetric flow rates or highly polluted wastewater |
Nanofiltration | Nuisance from odor is lowHigh rejection of organic compared to ultra-filtration.Hardness removing up to 50%, up to 90%, and almost all turbidity in wastewater.Generate a lot of concentrated effluents which increase environmental pollution | Wide application in many industriesLow energy requirementMore economic compared to reverse osmosisLow operating pressureThe ability to recover metals from wastewater | Public awareness is neededNeed skilled labors | Fouling problemsLow performance at high temperature |
Waste stabilization ponds | Nuisance from odor is high | The process takes a long time and a large area | No need for skilled labor | A long time of treatment |
Constructed wetlands (CWs) | Nuisance from odor is high | Inexpensive, easy to operate, and maintenance |
Table 15: A comparison between different wastewater treatment methodologies (Arora and Kazmi., 2015; Lourenco and Nunes., 2017). This table gives an idea on the environmental, economic, social and challenges of each process.
Integrating circular economy principles in sewage treatment enhances sustainability by promoting the reuse of treated water, protecting ecosystems and fostering resilient wastewater management. Reusing treated wastewater is crucial for augmenting water resources and minimizing the environmental impact of untreated disposal (Pereira et al., 2002; Massoud et al., 2018). While agriculture presents the greatest potential for reuse, treated wastewater also supports commercial, industrial, environmental, recreational, and groundwater recharge sectors. Implementing xeriscaping with drought-resistant plants and efficient irrigation reduces water demand, while harvesting and treating stormwater for non-potable uses mitigates runoff and maximizes rainwater as a resource.
8. Conclusion: The Importance of Making Informed Choices
The evolution from basic sewage treatment to advanced methods reflects our commitment to sustainability and environmental stewardship. Integrating renewable energy, leveraging advanced technologies, and focusing on a circular economy highlight our innovative approach. Effective treatment, minimal environmental impact, resource recovery, and water conservation are crucial. The future of wastewater treatment will prioritize sustainability, energy efficiency, and technological advancements to meet these goals.
8.1. Summary: Understanding the Different Types of Sewage Treatment Plants
Grasping the various types of sewage treatment plants is crucial for mastering wastewater management. Each treatment method, from traditional primary and secondary processes to advanced technologies like membrane bioreactors and UV disinfection, serves a specific purpose. While chemical methods target particular pollutants, biological treatments utilize microorganisms. Integrating smart technologies and renewable energy enhances sustainability and efficiency. This overview sheds light on the evolving nature of sewage treatment, emphasizing the importance of innovative solutions for addressing environmental challenges and ensuring effective water resource management.
8.2. Call to Action: Address Your Sewage Treatment Needs Today
To effectively address your sewage treatment needs, evaluate your requirements for capacity, effluent quality, and regulatory compliance. Consult wastewater treatment experts to identify suitable technologies, prioritizing energy efficiency and sustainability. Develop a comprehensive budget that covers initial costs, ongoing expenses, and potential funding sources. Ensure compliance with local and federal regulations and engage the community to foster support for responsible wastewater management. Create a plan for transitioning to advanced treatment technologies, establish a maintenance schedule, and adopt sustainable practices like water reuse and energy-efficient systems. Stay updated on technological advancements to continually improve your sewage treatment processes, promoting community health and environmental preservation.